4 Diffusion and Osmosis
Introduction
The ability of cells to move molecules in/out of their plasma membrane is critical to their survival. This means that as a multicellular organism, your survival is also dependent upon this molecular movement. At the same time, it is equally vital to be able to prevent the passage of certain molecules into or out of the cells. Thus, the cell membrane serves as a gateway through which all substances must pass when traveling into or out of the cell. How does the cell control this movement? To understand this process, we must first discuss what causes molecules to move in the first place.
At any temperature above absolute zero (-273 °C; the temperature at which molecular movement ceases), all molecules are in motion. This is because all molecules contain energy, and this energy causes them to vibrate and jiggle about. Adding more energy causes the molecules to increase their motion. Think about an ice cube. At low temperature, the water molecules are frozen in a crystalline matrix. Even though they are in a solid state, the molecules are still vibrating within that matrix. If you add heat (a form of energy), what happens to the ice? As the molecules absorb the heat energy, they increase their movement and begin to bump and slide past each other. This is what happens when ice changes states from a solid to liquid water. What if we keep adding heat energy? The molecules move more and more, bumping and pushing each other until they force themselves to spread out and the water turns to a gaseous vapor.
Clearly, individual molecules are too small to see with the naked eye. However, as molecules move they bump into other material. We can actually observe this molecular movement in the form of Brownian motion. Brownian motion is the movement of small particles due to impacts from molecules moving around them.
A solution is a mixture of substances in which a minor component (solute) is dissolved in a major component (the solvent). If you start with a pure solvent like water, and add some solute (e.g., a sugar cube), molecular movement causes the solvent molecules to bump into the solute molecules (think Brownian motion), causing the solute to spread out. This spreading of solute molecules is an example of a process called diffusion. Diffusion is the movement of any substance from an area of high concentration into an area of low concentration. Eventually, the solute will completely dissociate from its concentrated form and spread out evenly within the solvent. At this point the solute has reached equilibrium within the solvent.
Osmosis is a specific kind of diffusion in which water moves across a semipermeable membrane from an area of high water concentration to an area of low water concentration. A semipermeable membrane is a barrier that allows the passage of some substances but not others. In the case of osmosis, the semipermeable membrane allows water to pass through, but not solutes. For example, let’s say we have two solutions separated by a semipermeable membrane. Solution 1 on the left is composed of 90% water and 10% table salt. Solution 2 on the right contains 70% water and 30% table salt. If the membrane is permeable to water, but not to solutes, which way will the water diffuse?
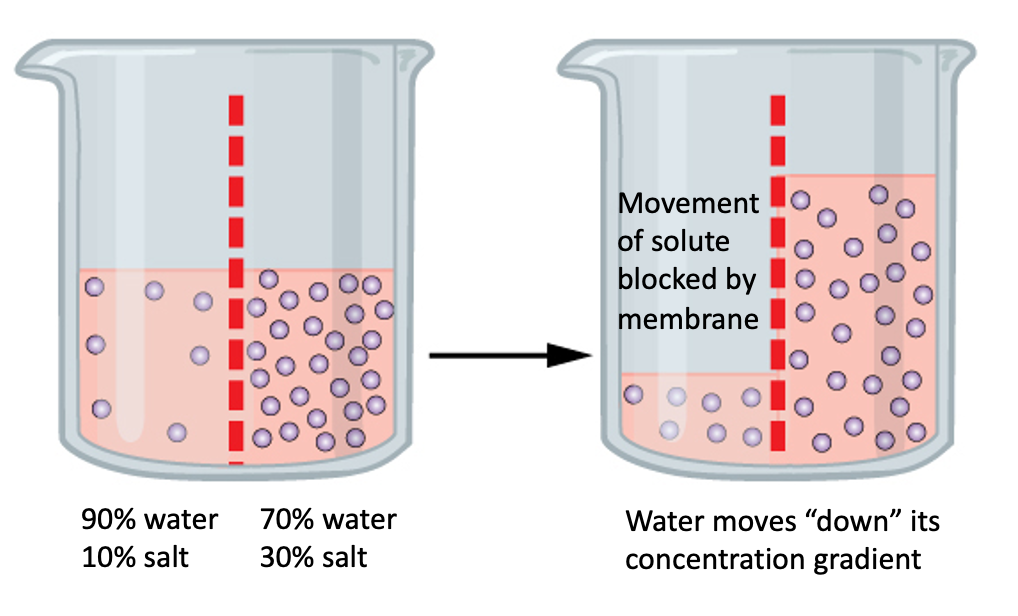
Since diffusion involves movement of a substance from an area of high concentration to an area of low concentration, the water will diffuse “down” its concentration gradient, from Solution 1 into Solution 2. Which way will the salt move? Since the membrane is impermeable to solutes, the salt won’t move at all.
At the start of this process, Solution 1 has a higher concentration of water, and a lower concentration of salt than Solution 2. Thus, water diffuses out of Solution 1, into Solution 2. At the same time, water “wants” to move from Solution 2 into Solution 1, but due to the concentration gradient (of water), it cannot. We use the prefixes hyper (more), hypo (less), and iso (same) along with the root terms osmotic or tonic to describe the relationship between solutions separated by a semipermeable membrane. The amount of pressure needed to stop osmosis from taking place is called osmotic pressure. In the scenario above, it takes more pressure to stop water from diffusing into Solution 2 than into Solution 1. Thus, Solution 2 is hypertonic (hyperosmotic) relative to Solution 1. Solution 1 is hypotonic (hypoosmotic) relative to solution 2.
Over time, as Solution 1 loses water and Solution 2 gains water, the concentration gradient between the solutions will decrease. When both solutions reach the point where their water concentrations are equal, the solutions have reached equilibrium. At this point, both solutions are isotonic (isosmotic), and water passes freely between the solutions.
So, how does all of this diffusion and osmosis stuff pertain to living systems? Cell membranes are made up of a bilayer of phospholipid molecules. Phospholipids have a polar head (containing phosphates) and two non-polar tails (composed of fatty acids). The non-polar tails are hydrophobic (water hating), and because of this are driven away from the aqueous (water based) solutions inside and outside of the cell. The polar phosphate heads are hydrophilic (water loving), and they are attracted to water. This causes the phospholipid molecules to form into a complex with a non-polar internal layer encased between two polar layers. This bilayer forms the basic structure of all cellular membranes.
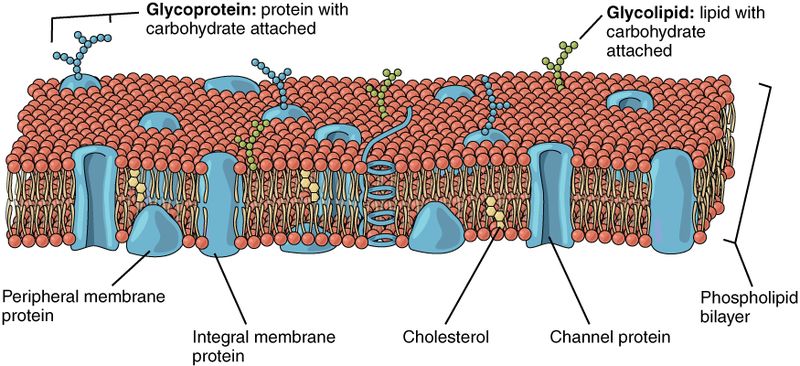
Gasses such as O2 and CO2 can diffuse directly across cell membranes. However, polar molecules like water and ions, or large nutrients and energy containing molecules, like glucose, cannot. Instead, these molecules must pass through channels in the membrane formed by proteins. Since protein channels only allow specific molecules to pass, the combination of phospholipids and transmembrane proteins makes the cell membrane semipermeable.
There are four ways that substances can be transported across the cell membrane.
- Simple diffusion through the phospholipid bilayer
- Facilitated diffusion through transport proteins
- Active transport through transport proteins
- Movement via vesicles (endocytosis and exocytosis)
Simple diffusion and facilitated diffusion take place passively as molecules move “down” their concentration gradients from areas of high concentration into areas of low concentration. By contrast, active transport uses energy to move molecules “up” their concentration gradient from areas of low concentration into areas of higher concentration. These processes are often during metabolism, including photosynthesis and cellular respiration.
For this week’s lab, we are going to focus on the process of osmosis and explore how concentration gradient affects this process. During this lab you will follow the scientific method to:
- Develop experimental hypotheses and predictions to explain the consequences of osmosis in animal cells
- Predict the direction water will move when given new biological scenarios
- Interpret data from a scientific article exploring the impacts of high solute concentrations on amphibians
Exercise 1. Osmosis in animal cells
Today you will use an egg membrane to model how osmosis works in animal cells. The egg you are observing was soaked in vinegar for ~48 hrs, which dissolved the egg shell. This “naked egg” now shows you the egg membrane, a layer of keratin proteins that separates the egg contents from its environment. The naked eggs were then subjected to two different treatments: exposure to corn syrup (25% sugar content) or exposure to distilled water. As you view the eggs, you’ll answer the following questions in your workbook:
- Why is the naked egg that was soaked in vinegar bigger than a shelled egg?
- What changes do you observe in the naked egg that was soaked in corn syrup?
- Draw a diagram of the egg in the corn syrup. Show the net direction of osmosis (the movement of water molecules across the membrane). Use your diagram to indicate why the egg in corn syrup changes shape and weight.
- What could you do to return the corn-syrup soaked egg to its original form?
- What changes do you observe in the naked egg that was soaked in distilled water? Does the egg soaked in water change shape and weight?
You’ll then complete practice problems to predict the direction of osmosis when given new scenarios.
Exercise 2. Osmosis in plant cells
Plants rely on water to maintain their structural rigidity. What happens to a plant when you don’t water it for several weeks? Clearly, it wilts. In plants, an organelle called the central vacuole functions to take up and hold excess water in the cell. The expansion of the central vacuole exerts pressure on the cytosol and presses the cell membrane against the cell wall. The force exerted by the water inside the cell against the cell wall is called turgor pressure. This force helps the plant remain upright. When the plant loses water, the central vacuole shrinks, turgor pressure is reduced, and the cell becomes flaccid. If the plant loses too much water, the plasma membrane may separate from the cell wall. This separation is called plasmolysis. We can cause plant cells to lose water by placing them in a hypertonic (hyperosmotic) environment.
If we immersed the plasmolyzed cells in a hypotonic environment, they would take up water and regain turgor pressure. However, the cells lose water faster than they can take it in, so this process takes longer than we have time available in lab.
Procedure
- Obtain a fresh leaf from the supplied Elodea plant, a clean slide, and a coverslip.
- Place the Elodea leaf on the slide with a drop of pond water and cover with the coverslip.
- Observe the plant cells under normal (isosmotic) conditions, and draw them in Figure 4.4.
- Add 1 or 2 drops of 20% NaCl solution to the edge of the coverslip.
- Observe the cells under the hypertonic conditions, and redraw the cells after a few minutes. Note your conclusions in the figure caption below.
Exercise 3. Article analysis: The impact of osmosis on amphibians
As your final exercise today, you’ll analyze a scientific article. Scientific articles are how scientists share their results, design their experiments, and learn about new scientific discoveries.
IMPACTS OF ROAD DEICING SALT ON THE DEMOGRAPHY OF VERNAL POOL-BREEDING AMPHIBIANS
Nancy E. Karraker,James P. Gibbs,James R. Vonesh
Before lab, you’ll prepare by just reading the abstract of the article. The abstract is posted on Canvas for you. During class, your group will analyze the results of this article and record your analysis in your lab workbook.